Taking the sun from under your nose
One of the benefits of installing your own solar panels is being able to do what you like with excess power. Phil Kreveld looks into how that might not be the case with the new legislation.
Significant electrical distribution developments are happening, but they’re going unnoticed. Well… not completely unnoticed as some irritated solar system owners will attest.
ADVERTISEMENT
Some are getting a surprise when they find out that their investment, which comes with the expectation of saving additional money on their electricity bills, is somehow being taken away.
Instead of cutting down on electricity bills from feed-in tariffs (miserably low these days) or somehow being able to continue charging batteries, they’re experiencing ‘export restrictions’ limiting the power available from their inverters.
That’s not all when it comes to ‘significant developments’. Back on 16 November 2022, SA Power Networks (SAPN) cranked up the low voltage network to above 257V, single phase – deliberately – to turn off solar inverters. In other words, SAPN made sure that the anti-islanding feature that all inverters must have, worked to its advantage.
The real reason for doing so was to preserve frequency stability in the state’s electricity system. There were some silly explanations from the premier and the energy minister along the line of “we were just producing much more energy than we could use” but in fact, the state’s connection to the main source of constant voltage and frequency, the Heywood interconnector with Victoria, had been lost.
Without that source, all those domestic grid-following inverters were not able to operate stably. The South Australian event is a harbinger of more occurrences like it and not only for that state, although its reliance on more renewables than elsewhere, sets it up for repeats given the state’s paucity of synchronous generation capacity.
The significant change in distribution networks is that they are becoming increasingly dynamic as a result of the growth in rooftop solar. It’s currently at about 14GW aggregate capacity and forecast by AEMO’s integrated systems plan to be 80GW by 2050.
Restricting solar export is a short-term fix and much more re-engineering will be necessary in order to integrate distribution networks with high penetration of rooftop solar into the overall grid. Export from solar systems is being restricted to prevent reverse power flow which is responsible for higher than wanted voltages. Voltage control at substations is achieved by on-load-tap changers (OLTC) on the main transformers.
As the medium voltage feeders, usually 22kV, to the low voltage edge of the network become lightly loaded due to large solar export, tap changers run of out range in an attempt to bring the voltage back to between acceptable high and low limits.
The problem around the corner is that with domestic, commercial and industrial solar systems being counted on as contributing the order of 30% of total demand by 2050, even with increased consumption patterns, there will be reliance on exported power, even if the legitimate question is what happens to the excess.
The answer: storage for cold start generators required for service in blackouts.
Already, AEMO is investigating fast frequency compensation ancillary services (FFCAS) whereby new forms of aggregation will provide frequency support within a 100ms timeframe through solar and battery power injection. Since 2016, distribution networks have been experimenting with virtual power plants (VPP) which essentially also provide frequency stabilising services.
In short, there is a clash between what distribution networks would prefer (no power export) and what is being counted on as stabilising energy from rooftop solar systems.
Presently, some distribution voltage control is achieved via lagging reactive power (power factor control) from inverters. They can also provide leading reactive support to increase voltage if required. Due to low inductive to resistive impedance ratios (X/R) of feeders, the effectiveness of reactive power voltage control is limited, and reducing the real power available from inverters represents the only practical method.
AS 4755 specifies the inverter control by means of demand response enabling devices (DRED) capable of providing directly or indirectly, for example via a smart meter, the operating instructions to inverters as shown in the tabulation.
Controlling power export, or in other words, simply controlling inverter power, requires the control of inverter AC current as we are always dealing with grid-tie inverters. Assuming the current to be in phase with line voltage at the point of inverter connection, the power delivered to the connection point is where Vgm and Im are the maximum values of the grid voltage and inverter current sinewaves, and it is assumed that the inverter current is in phase with the grid voltage (in order to provide maximum power).
The solar inverters in distribution networks are grid-tied, voltage source inverters were supplied DC power through maximum power point trackers (MPPT) to provide maximum AC power (at least that was the case in versions preceding AS 4777.2).
MPPT operate via hill-climb, conductance minimum or more complicated fuzzy logic suitable for multi-panel orientations with several or more local power maxima. The open circuit condition of the solar panels yields maximum voltage and hill-climb evaluates power increments, dp, equal to di. dv steps, in search for the maximum of V.I. Output current from the inverter is thus completely dependent on the DC power provided via the MPPT.
Once the controlled current mode is established, MPPT cannot function and this can lead to problems when the controlled current mode is relinquished because many solar installations have minor and major maximum power points with the possibility of the MPPT settling on a lower maximum. Basically, the domestic and smaller commercial and industrial inverters are single phase limiting power to 10kW but domestically half that rating applies as a maximum.
Current control in voltage-sourced inverters is achieved by varying the modulation index of sinewave pulse width modulation (SPWM) as shown in Figure 1.
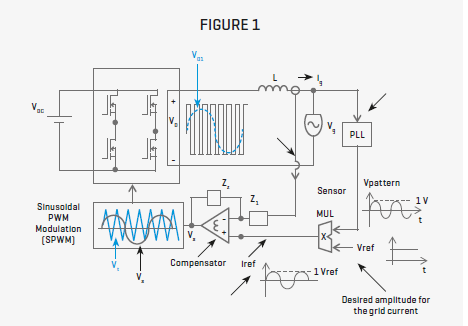
Figure 1: Schematic representation of a grid following, grid-tie inverter. The carrier frequency (in blue) intersects with the sinewave generator to produce a series of pulse-width modulated pulses whose higher frequency harmonics are attenuated by the inductor, L, in the inverter output. The sinewave generator, via the phase-locked loop (PLL) and current sensor, H, and compensator provides in-phase current with respect to grid voltage, Vg
The sinewave generator intersects the triangular, high-frequency carrier to generate a series of pulses for which the first harmonic is the fundamental frequency of 50Hz.
The maximum modulation index is achieved by adjusting the sine wave peak to the peak of the triangular carrier amplitude. For a DC link voltage of 200V, the peak voltage is ~325V peak and the RMS value is 325/Ö2 or 230V (note that 200V, through switching of polarity provides +200 for positive sinewave excursions and -200V and negative ones).
We are dealing with voltage-sourced inverters and in order to push current out into the network the inverter voltage has to exceed that of the grid, therefore requiring an impedance-offering element, usually an inductor, between the inverter and the point of connection, the inductor is normally an integral part of the inverter.
In Figure 2, peak values of the sinewaves rather than RMS are used because it focuses attention on the maximum voltage available from the inverter DC link. X is the impedance of the inductor in ohms at 50Hz. High-frequency harmonics resulting from the triangular carrier frequency, typically several kilohertz, are attenuated by the inductor because its impedance rises linearly with frequency.
The phasor diagram shows a right-angle triangle because the assumption has been made that the inverter current is in phase with the grid voltage to provide maximum power. On that basis, Em is given by.
The maximum current that can be drawn is determined by the energy stored in the DC link capacitor of the inverter (see Figure 1) which is replenished on each ‘beat’ of the carrier frequency by the MPPT or it’s a smaller value as commanded, for example, by an operating instruction (IO) as shown in the table above.
Current (and export current) is controlled via a multiplier connected to the phase-locked loop (PLL) of a grid tie inverter (refer to Figure 1). The PLL is essential for grid tie inverters by ensuring that the current bear a fixed phase relationship to the grid voltage.
The PLL follows the grid voltage and its output is sinewave in phase with it. As is shown in the phasor diagram (Figure 2), the modulating voltage Em, is ‘advanced’ in phase angle, but it tracks the PLL. The phase angle is given by so that both the current amplitude and the grid voltage affect the phase angle. The actual control is achieved by a proportional-integral-differential (PID) feedback controller. The multiplier referred to above receives an input refence value equal to the desired current if current control is required.
In power export limiting, we have seen that it’s given by replacing with
. The internal load consumes
where, for clarity the inverter current is distinguished from export current with the subscript, inv.
As for real power, the above expressions are correct. But what happens if the internal load has a power factor less than unity? Power consumed is given by the general formula, where cos φ is the power factor and V and I are RMS values.
Under normal operation, grid-tie inverters operate at unity power factor. Therefore, the reactive power, VI sin φ has to be supplied by the grid. Consequently, if a current transformer is being used to measure the current ‘flowing towards the grid’ on the service line connecting the installation to the street, the total current is equal to where all currents are RMS values and IL is the internal load current for the installation.
There is no ‘subtraction’ of the reactive current because it at 90° to exported current. The ‘addition’ of the reactive current is shown in Figure 3.
If, for example, the internal load has a power factor of 0.90 the reactive component is 0.436. It will therefore be clear that power measurement rather than current measurement works to the advantage provided the same in-phase current component underlies the power measurement. A two-element smart meter would be a good solution for the task of export control.
It’s therefore better to measure actual useful power rather than current (by means of a current transformer). As to current measurement, distribution networks might very well argue that being left to supply reactive current ‘only’ isn’t fair in terms of recouping network charges which for ‘bundled’ consumers are based on a portion of energy (i.e. real power-unit time product) delivered.
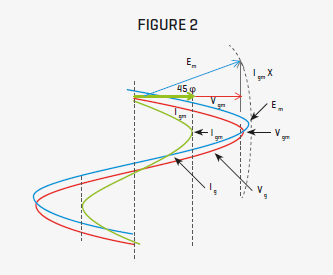
Figure 2: The phasor diagram showing the magnitude and phase angle relationship between the modulated voltage, Em, and grid voltage, Vgm, to keep the current in phase with the grid voltage (as shown by the sinewaves).
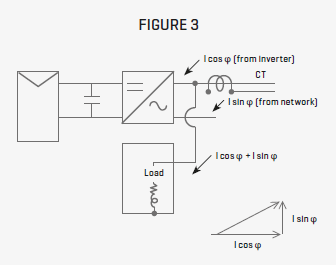
Figure 3: Shows a grid-tie inverter supplying a load with a power factor less than unity. The inverter supplies the power needed by the load, I cos φ but the reactive current, I sin φ is supplied by the distribution network. The CT measures the total current Ö(I2cos2φ +I2sin2φ).
Some inverter manufacturers have built-in smart meters, usually two-element, bi-directional. Most meters interface via Modbus, RS485 (an industrial-grade serial link), IIoT or WiFi, however, the bulk of inverters installed some years ago require the installation of a CT (or preferably, a two-element, bi-directional smart meter for power export control).
So where to from here? An export limitation is in many ways a patch-up solution and doesn’t allow the full advantages offered by the distributed energy resources in distribution networks.
Under strong midday insolation, many distribution networks are virtually independent of the transmission grid. Because the inverters are grid following a source of stable voltage and frequency has to be available but that doesn’t imply that a large degree of independence, as well as complete independence, isn’t possible.
Figure 4 is an extreme simplification in which all solar inverters have been aggregated into one current generator, I. The use of this term may be confusing as the grid-tied inverters are voltage sourced, although with controlled current.
Viewed from the network connection point towards an inverter it appears as current generator because it does not, by and large, affect the voltage at the connection point. The source of voltage for the inverters is shown as EÐd1, i.e.: its phasor angle is d1. The load for the network has been aggregated as P and reactive load Q. However, if as in the diagram below, the load, P, is taken care of by current generator, I, then generator is there to supply Q, i.e., reactive power only.
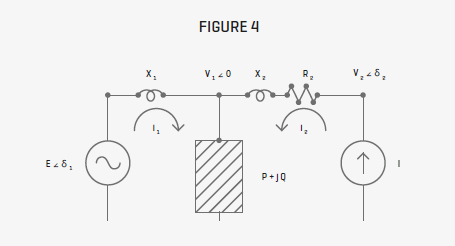
Figure 4: A highly simplified representation of a ‘self-supporting’ (almost) distribution grid with sufficient grid-tied solar inverters to at times supply the real power demand, P. The reactive component, Q is supplied by the synchronous generator, shown on the left.
With appropriate design of storage and voltage-forming inverters supported by storage batteries and charged by the grid-tied solar inverters, the voltage forming inverters would supply a stable voltage and frequency to the grid-tied inverters.
The technology for doing this is there, but it remains to be tried in practice. The Power of Choice requirements that separate the supply of revenue meters from services relating to meter reading and meter installation and the siloing of metered data information is handicapping the use of metering for not only inverter power export control but also for intelligent overall grid control.
What is clear is that not making full use of the advantages provided by rooftop solar is unwise. Our problem is that the distribution networks to which we attach the millions of rooftop solar systems were not designed for that purpose.
What really needs to be done is a comprehensive redesign, very likely a ‘system’ design, not only changing hardware, for example swapping transformers for smart transformers that allow very flexible use of solar resources, but new distribution-based control systems.
-
ADVERTISEMENT
-
ADVERTISEMENT